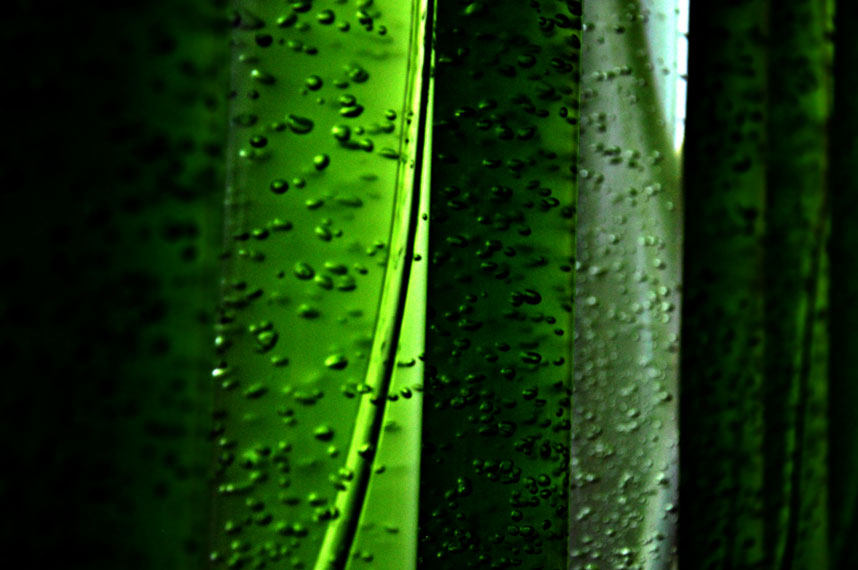
Algae Photobioreactor
Summary
A photobioreactor is a vessel in which photosynthetic organisms are grown, using light and various nutrients. Commercially, algae and cyanobacteria may be grown in a photobioreactor to produce oils which may be converted into biodiesel fuel. This module can be used to teach students about design principles and optimization.
General Information
Bibliography
- Ugwu, C.U., H. Aoyagi and H. Uchiyama. “Photobioreactors for mass cultivation of algae.” El Sevier (2008): 4021-4028.
Materials & Methods
In the case of a reactor design project, the students will decide the amount of growth medium, algae, and what type of container from which they would like to build their PBR.
- Reactor Container (i.e. soda bottle, pie tin, plexiglass, tupperware etc.)
- Light source. We simply use fluorescent lights from Home Depot during outreach events, but we use red and blue LEDs in our lab.
- Sparger. Bubble stones for fish aquariums work well for this purpose. If you wish to make a line or wall of bubbles, pet stores also sell flexible bubble tubing.
- Aquarium air pump and/or water pump, and the associated tubing.
- Nutrients (Miracle Gro plant food or other commercial fertilizers will work)
- Tap water
- Algae. A sample may be collected from most stagnant bodies of water, or you can buy live algae samples online (e.g. fishersci.com, Carolina Biological Supply).
- A means to measure algae concentration. For this task a cell count from a microscope image will work, or a measure of optical density from a spectrophotometer will also work. We have a home made spectrophotometer module that we have found to work great for this task.
- Miscellaneous – Basic prototyping tools are needed, such as a variety of adhesives, and cutting tools.
Background for Teachers
Microorganism growth
Single celled organisms, such as cyanobacteria, grow and reproduce like most any other organism. They consume sources of energy, and convert that energy to produce the biological molecules needed to maintain themselves and to create the biomass needed to assemble offspring.
Figure 1. The various phases of a typical microorganism growth in a batch system.
If we were to make up a solution of nutrients in a container and introduce microorganisms that could use those nutrients, we would likely see a very typical pattern of growth (Figure 1). At early times, the organisms may find themselves in an environment that is slightly different to the one in which they were growing in terms of several factors, such as temperature, pH, nutrient concentration, and so on. As the organisms retool and grow accustomed to their new environment, very little growth occurs; we call this period the lag phase. However, once they are acclimated, they enter the exponential growth phase. On the scale of hours, 1 cell quickly becomes 2, 2 becomes 4, 4 becomes 16, 16 becomes 32, and very quickly thousands of organisms can become billions (which is why the y-axis in Figure 1 is the logarithm of cell concentration). Once nutrients are used up and/or toxic byproducts of metabolism build up, the death rate of the organisms begins to match their growth rate. Living cell can use the nutrients liberated at their cohort’s death and that way maintain a stable population. We call this phase the stationary phase. Eventually toxic byproducts build up to an unmanageable level and the death rate surpasses the growth rate, in the death phase.
Within the photobioreactors built in this module students should expect similar stages of growth.
Algae
Figure 2. Synechococcus Elongatus, the blue-green algae used in our reactors.
The term algae can mean several things. It may refer to multicellular macro algae, such as massive sea weeds, or it may refer to microscopic single-celled plant life, such as diatoms. However, in photobioreactors, we are mainly interested in growing blue-green algae, which are not members of the plant kingdom at all; they are bacteria, cyanobacteria.
Algae of all sorts may be found in our oceans and other bodies of water. Anyone who has owned a fish tank knows how persistent algae can be. Algal blooms are a phenomenon where a body of water, whether it be freshwater or marine, becomes oversaturated with algae species. Sometimes, depending on the type of algae there exists a color difference in the water. When there is an over saturation, cyanobacteria (blue-green algae) exhibits a green color and is mainly found in freshwater sources while phytoplankton shows a red color (named red tide) and is found in marine and coastal environments. They are harmful to the environment because some algae sources excrete toxins which can kill aquatic life. Also, with the mass amounts of algae, much of the oxygen in the water is used as a food source by the algae when there is no light for photosynthesis. This drives aquatic life away from the area. But how can we use such a force of nature to our benefit?
Photobioreactor Design
For many years, photobioreactors (PRB) and the algae produced within have been of significant interest for their potential in the food industry, environmental cleanup, and as sources of cleaner energy, among other things. Algae, in the wild, have been used for water treatment and even clean up of oil spills due to their ability to use their surroundings as food and turn it into biomass. Some species of algae and bio-organisms secrete a biogas in the form methane and other gases that could potentially be captured and burned as fuel.
There are a vast number of different styles of photobioreactors that have been tested in research. The ultimate goal of these tests is to create a vessel that will combine all of the important variables needed to supplement the overall algae yield. Important variables to make the most effective environment for algae are as follows:
- Photonic concentration (solar or artificial) – Photosynthesis within algae must occur to create the sugars, oils, and other molecular building blocks needed to sustain their life. Simply, algae need light (specifically and most often red and blue wavelengths).
- Nutrients – While the majority of biological molecules are made up of atoms of carbon hydrogen and oxygen, which algae can find in water and carbon dioxide, many other important atoms are still needed for growth. Just to name a few, algae need nitrogen, phosphorus, iron, calcium, and more. These micro and macro nutrients are typically dissolved in water to make a nutrient media for the algae.
- Dissolved gases – Algae need carbon dioxide, as well as light to perform photosynthesis. They also need oxygen when it’s dark and they must burn the sugars made during the day. To keep the algae breathing properly in a photobioreactor, either air must be bubbled through the media or the liquid media must be made to flow past air.
- Mixing – Algae will settle if in stagnant water and the growth of those at the bottom, away from the light, will be inhibited. To avoid stagnant regions the fluid in the photobioreactor should be in continual motion.
- Temperature – All organisms have some range of temperatures outside of which they cannot live or reproduce. Most algae can do fine at room temperature but below freezing and above 35°C your photobioreactor is not likely to be productive.
The best combination of these aspects will ensure a healthy crop of algae and therefore a better yield.
Figure 3. Examples of possible bioreactor designs.
Two categories of photobioreactors exist, one being an open system PBR and the other being a closed system PBR. Benefits of an open system lie in the ease of construction. An open system can be in the form of a lake, pond, or some large open vessel that can hold the water. The downside of this design is in its susceptibility to evaporation and also contamination by invasive species. Once the algae flourishes, it is also difficult to get the maximum amount of light to all the algae since the pools are somewhat deep and efficient circulation is problematic.
An example of such a PBR is show in Figure 3A. Students could make sure PBRs in a baking pan, for instance, but they will need to give some thought to how they will keep the fluid in motion without creating stagnant zones, where the algae builds up.
Closed systems, on the other hand, are much easier to manipulate leading to the optimization of essential variables in the system. Agitation of the system can be easily implemented which will prevent the algae from sticking to the walls of the vessel and diminishing the amount of available light. The temperature of the system can also controlled with much more accuracy using a heating jacket or some kind of similar technology.
A few examples of closed systems can be seen in Figure 3B-E. The flat plate PBR will maximize the surface area of the algae to get the most light energy for photosynthesis (Figure 3B). Vertical column PBR’s have the luxury of air-lift mixing of algae solutions by using materials such as spargers (Figure 3C), or a column with a thicker diameter can be used with a rotating propeller (Figure 3D). And lastly, internally illuminated photobioreactors are able to give light to the algae from two directions and can be integrated a with a mixing system to keep the solution well mixed. Figure 3E shows such a system where the reactor has an annuls in which a light source is placed. Mixing in this system is caused by air lift. Bubbles from the circular sparger near the annulus entrain and pull liquid up with them; once the liquid reaches the surface of the PBR, it then must flow down the outer radius of the PBR in cyclic motion.
Instructional Procedures
- Students should be introduced to the importance of algae research and given a basic understanding of what is needed to grow algae efficiently, as described in the Teacher’s Background section of this module.
- Teams of students should then be set to the task of designing their PBR on paper. They should show consideration of design objectives and detail the materials they will need.
- Materials may be provided or they may have to work with what they can find around their house.
- Once the needed materials are gathered, teams should then build their designs. If they do not think about it, the instructor may want to remind them that sterilization of any material that will come in contact with the algae may be important; this may be done with isopropanol or ethanol, as long as time is given for it to dry before the algae is introduced.
- Each team should be given the same starting solution with the same concentration of nutrients and algae (though each reactor may use a different volume of the solution). For this teaching module we have found that solutions of 200 to 800 mg/L of Miracle Gro in water work effectively. At this point the solution may be boiled to sterilize, but if you are using distilled or deionized water that shouldn’t be too much of a concern. To that solution add approximately 5% by volume of a live mature algae culture to act as the inoculum, the seed from which their algae cultures will grow.
Figure 4. Student-made PBRs.
- Each team should also have equivalent illumination. You can let these grow using daylight, but they will grow faster if you use constant artificial light, such as fluorescent bulbs or a large bank of LEDs.
- Note the liquid level height of each student’s PBR. The reactors should be judged on their ability to grow algae, not on how well they evaporate water. Thus, throughout or at the end of the growth period, water should be added to bring the level back up to its original level; this way all designs will be judged on a fair basis of algae concentration.
- Growth may be tracked in several ways. At the same magnification for each team, you may take count the number of cells in the image to qualitatively measure growth. If you have a hemocytometer you may obtain a quantification of growth with a microscope. We have used our home-made spectrophotometer to successfully track cell concentration, and a commercial spectrophotometer works as well.
- Leave algae to grow, checking every day to make sure that there is sufficient water and that nothing has gone wrong, such as leaks or failed pumps. You may also wish to measure growth more often to see if the growth curve matches that predicted by theory (Figure 1).
- Take final measurements of algae concentration and declare a winner.